Abstract: The integration of 5G core networks with edge computing marks a transformative advancement in telecommunications, enabling high-speed connectivity with ultra-low latency for modern applications. This article explores the design and deployment of 5G core networks at the edge, highlighting key technologies such as network slicing, SDN (software-defined networking), and virtualization to support latency-sensitive services. It emphasizes the importance of orchestration and automation in optimizing network operations and discusses the critical role of security in ensuring resilient deployments. Through real-world applications like autonomous vehicles, robotics, and industrial automation, the article demonstrates how 5G and edge computing create new business opportunities while addressing challenges related to energy consumption, network traffic, and complex deployments. As industries continue to adopt these technologies, the collaboration between 5G and edge computing paves the way for innovative solutions that enhance efficiency, scalability, and real-time data processing.
Keywords: 5G Core Networks, Edge Computing, Network Slicing, Software-Defined Networking (SDN), Network Virtualization, Orchestration, Automation, Low-Latency Networking, Real-Time Data Processing, Autonomous Vehicles, Robotics, Industrial Automation, Internet of Things (IoT), Energy-Efficient Networking, Digital Transformation
Designing and deploying 5G core networks at the edge with end-to-end networking is a crucial advancement in telecommunications, merging the high-speed capabilities of 5G technology with the low-latency benefits of edge computing. This integration facilitates real-time data processing and enhances application performance, which is essential for modern enterprises aiming to leverage digital technologies to improve efficiency and create new business opportunities[1][2].
The strategic deployment of 5G core at the network's edge allows for reduced latency and improved data throughput, thus enabling new services and applications across various industries[3].
Key design considerations for 5G core networks at the edge include the implementation of virtual network slices, which are vital for supporting latency-sensitive applications. The ongoing development of 5G standalone core infrastructures is essential for enabling these network slices to function effectively, ensuring that applications can operate without lag[1]. Security remains a significant concern, necessitating a comprehensive, multilayered defense strategy to protect against vulnerabilities in the network[4]. Edge computing's close proximity to radio access points further emphasizes the need for collaboration between network operators to maintain service efficiency[5].
The deployment architecture of 5G core at the edge is characterized by the strategic placement of network functions to optimize performance. This involves integrating network function virtualization, software-defined networking (SDN), and network automation to support edge computing capabilities[4]. This architecture allows for flexible geographic deployments, extending from co-located setups with the radio access network (RAN) to broader distributions, ensuring that computational resources are readily accessible[3]. By leveraging these technologies, businesses can enhance their digital experiences and efficiently manage data-intensive applications[2].
End-to-end network slicing, orchestration, and automation are pivotal in maximizing the potential of 5G and edge networks. These processes allow for the dynamic management of network resources, ensuring tailored services that meet specific application demands[6]. The collaboration between 5G and edge computing not only resolves challenges such as network traffic and bandwidth limitations but also paves the way for innovative use cases and industry applications, including autonomous vehicles and real-time robotics[7]. Despite challenges related to energy consumption and the complexity of orchestration, the evolution of 5G networks alongside edge computing heralds a promising future for connected technologies and digital transformation[2][8].
5G Core and Edge Computing
5G Core and edge computing are integral components in the evolution of modern networking, particularly as enterprises seek to harness the power of real-time data processing and improved application performance. These technologies work symbiotically, enhancing each other's capabilities to offer robust digital experiences and support data-intensive applications[1][2]. The 5G Core, a key element of the 5G network architecture, plays a pivotal role by functioning near the edge of the network, effectively bridging the Radio Access Network (RAN) and the broader IP-based internet[3][9]. This strategic positioning allows for reduced latency and increased efficiency, bringing compute capabilities closer to the end-user[2][3].
The 5G Core incorporates critical functions such as network function virtualization, software-defined networking (SDN), network automation, and support for IoT connectivity[4]. By integrating these functions, 5G networks can deliver the high-speed, reliable connectivity that modern applications demand. The integration of edge computing further enhances this setup by moving intelligent computations to the edge, allowing networks to react promptly and enable new real-time services[10]. As a result, businesses can leverage these combined technologies to innovate and streamline operations, paving the way for a more connected and efficient future.
Key Design Considerations for 5G Core at the Edge
The design of a 5G Core network at the edge requires a strategic approach to ensure optimal performance and scalability. One of the primary considerations is the implementation of virtual network slices, which necessitate a 5G standalone core. This infrastructure is still under development by many carriers, and its successful deployment is crucial for supporting new applications that are sensitive to latency and lag without the enhancements offered by 5G and edge computing[1].
Observability solutions that are simple to install, use, and scale are expected to play a pivotal role in the deployment of 5G Core networks. Approaches such as a federated mesh with a central Istio control plane are explored, although the unique architecture driven by 3GPP standards poses challenges in predicting service discovery outcomes[11]. The evolution of microservices has further introduced complexities in designing, implementing, and maintaining scalable 5G Core platforms. Solutions like the 5G Open HyperCore architecture leverage Kubernetes for edge applications, enhancing container observability with a service mesh[11].
Security is another vital consideration, requiring a multilayered defense strategy to address vulnerabilities across different parts of the core network. This strategy must extend beyond technical frameworks to include processes for identifying, preventing, and remediating vulnerabilities' impacts[4]. Moreover, security discussions often focus on the separation of RAN (Radio Access Network) and core network functions, guided by 3GPP standards and commercial deployments[12].
Edge computing is integral to 5G networks, emphasizing the importance of minimizing latency for applications like autonomous vehicles and robot control. The proximity to radio access points is crucial, requiring close collaboration with network operators to ensure service efficiency[5]. The deployment of 5G Core at the edge is thus a cornerstone of digital transformation, enabling real-time data processing and seamless integration of IoT devices and endpoints[2][13].
Deployment Architecture
The deployment architecture of 5G Core at the edge involves strategic placement and integration of network functions to maximize performance and meet specific use case requirements. The architecture and deployment can be customized for various scenarios to harness the full potential of edge computing[6]. The 5G Core, which serves as the backbone of the 5G network, must be strategically deployed to ensure reliable connectivity and optimal network performance[9]. Unlike its predecessor, the Evolved Packet Core (EPC) in 4G, the 5G Core (5GC) is designed to operate closer to the edge, bridging the Radio Access Network (RAN) and the broader IP-based Internet[3]. This flexibility allows for a range of geographic deployments, from co-located setups with the RAN to widespread areas spanning hundreds of kilometers[3].
A key element of the 5G Core architecture is its ability to support edge computing by virtualizing network functions and automating network operations[4]. Edge computing extends cloud resources and services to be distributed at the network's edge, enabling low-latency access to computational resources and data storage[7]. This architecture is crucial for scenarios requiring real-time data processing and high-speed application performance[1][2]. By bringing compute and storage resources closer to the data source, edge computing reduces latency and enhances the overall efficiency of the network[14].
Incorporating edge computing with 5G Core facilitates a symbiotic relationship, where both technologies enhance each other's capabilities[1]. The strategic deployment of 5G Core at the edge enables enterprises to leverage new digital experiences and support data-intensive applications by processing large volumes of real-time data efficiently[2]. This deployment architecture provides a robust foundation for emerging use cases and paves the way for innovative industry applications.
End-to-End Network Slicing
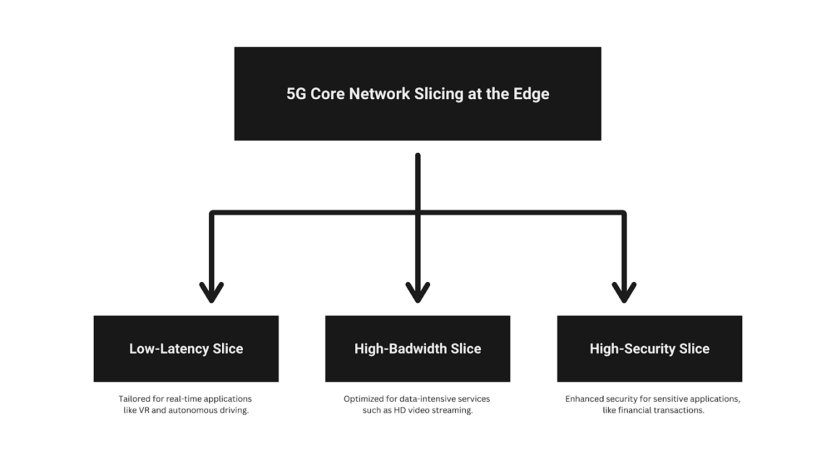
End-to-end network slicing in the context of 5G core deployment on the edge involves creating multiple virtual networks within the same physical infrastructure to cater to different use cases and service requirements. Each network slice can be tailored to specific needs such as latency, bandwidth, and security, providing a customized networking experience for different applications and industries[6].
The 5G architecture's flexibility allows for diverse configuration options of the network components, which is crucial for deploying network slices that can efficiently meet the varying demands of edge computing use cases[6]. For instance, latency-sensitive applications like mobile virtual reality (VR) and autonomous driving can benefit from the low latency and distributed nature of edge computing while also being supported by network slicing to ensure the necessary performance and reliability[5].
Network slicing in the 5G era is expected to work in tandem with edge computing to enhance overall network performance and provide robust support for the Internet of Things (IoT), software-defined networking (SDN), and virtualization[4]. These capabilities enable operators to manage network resources and deliver tailored services efficiently, thus maximizing the potential of 5G networks as critical infrastructure for the digital age[4].
Furthermore, deploying network slices at the edge can address some of the deployment challenges associated with 5G, such as network traffic and bandwidth concerns[7]. By strategically implementing slices at the edge, operators can improve cost efficiency and data security, ultimately enhancing the quality of service delivered to end users[7].
Orchestration and Automation
Orchestration and automation are critical components in the deployment and management of 5G core networks on the edge. The integration of these elements allows for the efficient handling of complex network functions, ultimately enhancing the flexibility and responsiveness of network operations. The use of sophisticated operating systems, such as Digi Accelerated Linux, plays a vital role in delivering advanced device functionality necessary for orchestration and automation[15].
The advent of 5G networks has introduced a paradigm shift in how networks are configured and managed. The automation capabilities enabled by 5G and other wireless technologies like Wi-Fi 6 allow for greater autonomy in applications such as vehicle systems and seamless workload migrations to the edge[14]. This flexibility is further bolstered by software-defined networking (SDN) and virtualization, which are essential in orchestrating network resources to meet varying demand levels and service requirements[4].
One of the key orchestration aspects is the ability to configure edge computing solutions dynamically. This involves setting up edge runtime infrastructure, network functions, and application functions tailored to specific use cases, as outlined in various proposed deployment options[6]. The integration of artificial intelligence (AI) with 5G further enhances orchestration by allowing models to be trained in the cloud and deployed at the edge, facilitating faster data rates and real-time decision-making processes[2].
However, the complexity of orchestrating and automating 5G networks at the edge also presents challenges, such as addressing network traffic, bandwidth concerns, and ensuring data security[7]. Despite these challenges, the gradual deployment of 5G infrastructure is expected to significantly advance the capabilities of orchestration and automation, paving the way for an ecosystem of new applications and improved service delivery[2].
Use Cases and Industry Applications

Edge computing, in conjunction with 5G core networks, is poised to revolutionize various industry sectors by offering enhanced capabilities and efficiencies. The implementation of edge computing features in 5G networks can significantly benefit industrial use cases by improving latency and providing a multitude of other advantages[6]. These improvements are particularly relevant for latency-sensitive applications such as mobile virtual reality (VR) and autonomous driving, where distributed functions close to the data source can optimize performance[5].
In manufacturing, edge computing is utilized to address specific use cases that require rapid data processing and analysis. This is accomplished through nonpublic deployment options and the strategic configuration of network architectures, enabling better performance and flexibility[6]. The partnership between technology giants like AWS and Verizon exemplifies how multi-vendor collaborations can enhance product interoperability and bring better connectivity to the edge, thus supporting complex industrial applications[14].
The vehicle industry is also experiencing a transformation due to the integration of edge computing and 5G technologies. For instance, the vehicular edge computing (VEC) architecture allows service providers to host services in close proximity to smart vehicles, reducing latency and improving quality of service (QoS)[7]. This advancement supports the development of better vehicle autonomy and the potential for workload migrations to the edge, further enhancing capabilities like smart vehicle communication and applications[14].
Furthermore, the rise of connected devices and the complexity of data generation necessitate robust monitoring and data management strategies. 5G networks facilitate real-time processing by distributing edge compute processing across the network, catering to unique application requirements in terms of storage, performance, and data integrity[13]. In enterprise settings, solutions like Aether, an open-source, Kubernetes-based edge cloud, exemplify how businesses can leverage 5G connectivity to support applications that demand low latency and predictable connectivity[3].
Challenges and Future Trends
The integration of 5G core networks with edge computing presents several challenges and promising future trends. As the number of connected devices and data-intensive applications grows, the demand for efficient and scalable network solutions becomes more pressing[2]. One of the primary challenges lies in managing the increased network traffic and latency requirements. Applications such as autonomous vehicles and real-time robotics require ultra-low latency, demanding the edge infrastructure to be as close to the radio network as possible[8].
Another significant challenge is network energy consumption, as the expansion of 5G networks could lead to higher carbon footprints. However, edge computing offers a potential solution by processing data closer to the source, thereby reducing the energy required for data transmission across the network[2]. The balance between deploying sufficient edge infrastructure to support these requirements and maintaining cost efficiency and energy savings is crucial[7].
Federating the telco edge remains an area of strong interest but is not without challenges. The practical implementation of federation among operators to provide seamless edge services is yet to be fully realized, posing questions on governance, interoperability, and standardization[2].
Looking forward, one of the promising trends is the symbiotic relationship between 5G and edge computing, which can significantly enhance application performance and real-time data processing capabilities[1]. This partnership is expected to drive innovation in various industries, enabling new use cases that leverage the strengths of both technologies[16]. As 5G networks continue to evolve, the customization of edge computing architectures to meet specific use case requirements will become increasingly important[6].
References
[1] Pare, D. (2023, April 19). What is the Relationship Between 5G and Edge Computing? Cradlepoint. https://cradlepoint.com/resources/blog/what-is-the-relationship-between-5g-and-edge-computing/
[2] Boyle, A. (n.d.). 5G and edge computing: Why does 5G need edge? STL Partners. https://stlpartners.com/articles/edge-computing/5g-edge-computing/
[3] Dryjanski, M. (2018, July). Chapter 2: Architecture. Systems Approach. https://5g.systemsapproach.org/arch.html
[4] Ericsson. (n.d.). 5G Core (5GC) network: Get to the core of 5G. Ericsson. https://www.ericsson.com/en/core-network/5g-core
[5] Irizarry, M. (2024). Securing the 5G Core: Challenges and Solutions. Palo Alto Networks. https://www.paloaltonetworks.com/cybersecurity-perspectives/securing-the-5g-core-challenges-and-solutions
[6] Kaji, H. S. (2021, September 29). Understanding 5G, A Practical Guide to Deploying and Operating 5G Networks, Edge Computing (Part 5). LinkedIn. https://www.linkedin.com/pulse/understanding-5g-practical-guide-deploying-operating-5-sadeghi-kaji
[7] Nar, F., Lajoie, E., & Lee, S. (2022, July 12). Edge computing: How to architect distributed scalable 5G with observability. Red Hat. https://www.redhat.com/architect/5g-core-observability-edge
[8] Teppo, P., & Norrman, K. (n.d.). Security in 5G RAN and core deployments. Ericsson. https://www.ericsson.com/en/reports-and-papers/white-papers/security-in-5g-ran-and-core-deployments
[9] Gulf South Towers. (2019, January 20). 5G and edge computing: Three key questions (and answers). Gulf South Towers. https://gulfsouthtowers.com/5g-and-edge-computing-three-key-questions-and-answers/
[10] Western Digital. (2019, May 23). Edge Computing: 5 Design Considerations. Western Digital. https://blog.westerndigital.com/edge-computing-5-design-considerations/
[11] 5G-ACIA. (2024). Industrial 5G Edge Computing – Use Cases, Architecture and Deployment. 5G-ACIA. https://5g-acia.org/whitepapers/industrial-5g-edge-computing-use-cases-architecture-and-deployment/
[12] Hassan, N., Yau, K.-L. A., & Wu, C. (2019). Edge Computing in 5G: A Review. IEEE Access, PP(99), 1–1. https://doi.org/10.1109/ACCESS.2019.2938534
[13] Bigelow, S. J. (2021, December). What is edge computing? Everything you need to know. TechTarget. https://www.techtarget.com/searchdatacenter/definition/edge-computing
[14] Remmert, H. (2021, March 19). What Is 5G Network Architecture? Digi International. https://www.digi.com/blog/post/5g-network-architecture
[15] Kinney, S. (2019, January 20). 5G and edge computing: Three key questions (and answers). RCR Wireless. https://www.rcrwireless.com/20190120/5g/edge-computing-5g-key-questions
[16] STL Partners. (n.d.). Edge computing questions and answers: What you need to know about edge compute. STL Partners. https://stlpartners.com/articles/edge-computing/edge-computing-questions-and-answers-what-you-need-to-know-about-edge-compute/
About the Author
Ishan Bhatt is an accomplished Network Engineer at Google, specializing in the design and deployment of advanced networking solutions for telecommunications, enterprise, and AI workloads. He focuses on implementing 5G core networks and edge computing to deliver low-latency, high-performance solutions for modern applications.